Discoveries take science by surprise
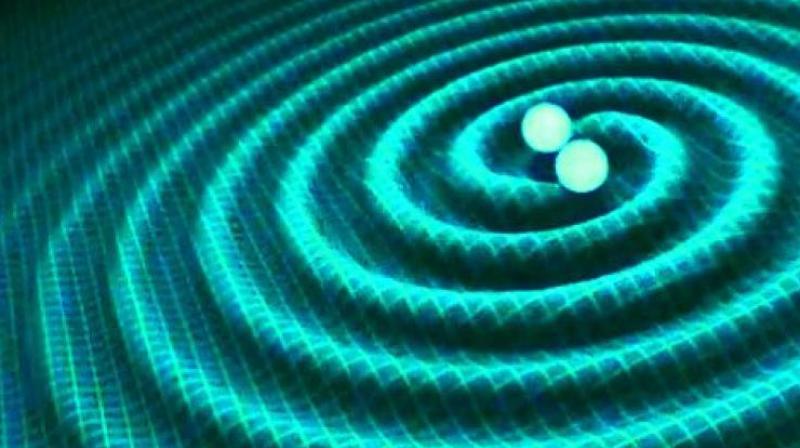
Waves are familiar to us from our daily experiences. Drop a pebble in the still water of a pond and you see waves emerging out from the point where the pebble hit the surface of the water. When we speak, our voice is conveyed from one point to another by sound waves. Unlike water waves, sound waves are invisible but their existence can be established by laboratory experiments. In a typical wave motion, disturbances go up and down such that the pattern repeats after a fixed distance identified as the wavelength and how often per second it does so, by its frequency.
A major progress in our understanding of what is light came with the experiments performed by Heinrich Hertz back in 1887. In the 1860s, James Clerk Maxwell had predicted that electrical and magnetic disturbances are interrelated and travel in the form of an electromagnetic wave. What we call light is a specific form of such waves with wavelengths between 400 to 800 nanometres. (A nanometre is a billionth part of a metre.) What about the waves whose lengths are beyond this range? Those which Hertz detected have wavelength of the order of metres and are identified with radio waves. Soon it became possible to identify the nature of various electromagnetic waves with their wavelengths.
These examples suggest that wave motion is ubiquitous. Does nature provide other examples of waves? What about gravitation? Although Newton’s law of gravitation had worked well for three centuries, cracks in its structure had begun to appear by the beginning of the 20th century. For example, Newton’s notion of instantaneous action was inconsistent with the newly established special theory of relativity proposed by Albert Einstein. Ultimately, rather than patch up the cracks, Einstein went for a completely new version of gravitation which he called the general theory of relativity.
Relativity theory is very elegant but at the same time, very unusual in structure. It is a remarkable combination of geometry and physics. The example of the Sun’s gravity will illustrate the basic idea. We start with the basic premise that in the absence of any gravitating body like the Sun, the geometry of space and time is Euclidean, that is the geometry formalised by Greek geometer Euclid; which we study at school. However, if the region has a massive body like the Sun, its gravitational effect would modify the rules of geometry of the region. An example is the rule we prove in our school geometry that the three angles of a triangle add up to two right angles (180 degrees).
If we station three observers A, B and C, say around the Sun such that they are able to exchange light signals between them, the signals AB, BC and CA form a triangle of light rays.
What is the sum of the three angles of this triangle? The general theory of relativity predicts that the three angles will add up to slightly more than two right angles. The excess will be of the order of a thousandth of a degree!
So small a result might be considered of little consequence. However, its existence or otherwise is of great significance for gravity theories. The above triangle measurement is impossible to make but a variation of it is possible. It measures by what tiny angle a ray from a background star is bent by the Sun’s gravity. This test favours the relativity theory and rules out the Newtonian law of gravitation.
The growing popularity of relativity also raised the question: Do waves of gravitation exist? Just as the electromagnetic waves travel with the speed of light and convey electromagnetic effects from one point to another, should we expect gravitational effects to be communicated by waves travelling with light speed? This was not an easy question to answer in view of the geometrical issues involved. The question remained unanswered during Einstein’s lifetime
But by the early 1960s, experts in general relativity were convinced that the above question had an affirmative answer. So the ball was in the experimentalists’ court with the challenge of detecting it. It was also clear that the overall effect to be observed was so small, one part in a thousand billion billion, that it would not be possible to have an Hertz-type experiment in the laboratory. So the eyes turned to the heavens where cosmic sources of such waves might exist.
A good source is in the form of binary stars. Two stars going round each other emit gravitational waves and these increase in intensity as the binary system shrinks and the two stars coalesce.
In the 1980s, there was indirect evidence for this happening. Radio astronomers Joseph Taylor and Russell Hulse found a binary system whose period of orbit was steadily decreasing. Indeed, a model of the system based on gravitational waves fitted observations exactly.
So here was a challenge for experimentalists to devise a direct way of detecting the above effect. Indeed, even earlier, since the 1960s, Joe Weber, a professor at the University of Maryland, had devised detectors of high sensitivity to detect cosmic gravity waves. But they were not sensitive enough. For, to measure and confirm the existence of these waves one has to measure the subtle changes of geometry taking place in a region through which a gravity wave has passed. The changes of length are estimated to be as small as one part in a hundred billion billion. Weber’s pioneering efforts indicated the need for a better technology. So it was decided to use very sensitive interferometers in which back and forth motions of lasers in high vacuum are used to measure length changes. Known as LIGO, for laser interferometric gravity observatory, two such detectors were sited diagonally across the United States with similar but smaller ones in Europe and Japan.
The patient efforts of experimentalists were rewarded about a year ago when a positive detection became possible in LIGO America. The data analysis suggests a source of binary black holes as massive as 25-30 solar masses revolving and coalescing eventually.
The main idea of the LIGO is ascribed to three US scientists — Rainer Weiss, Ronald Drever and Kip Thorne — but the overall credit is shared by over a thousand scientists. It is a matter of pride that Indian scientists like Sanjeev Dhurandhar and Bala Iyer in data analysis techniques and C.V. Vishveshwara in theory of sources have played an important role. Not surprisingly India is proposing to enter the LIGO field by erecting a replica of the US model. Happy hunting!